Biological Nanostructures and Nanomedicine
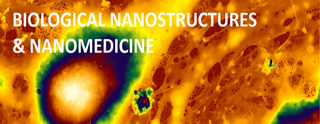
In the group research activities methods and tools of advanced nanotechnology are applied to investigations of biological structures at the nanoscale. Those nanostructures are monitored in live cells and tissues as well as in isolated molecular systems. The results are used for developing new methods of medical diagnostics, pharmacological drug testing, monitoring the toxicity of nanomaterials, and also for better understanding of basic processes responsible for the progress and therapeutic treatment of several civilization diseases.
Fundamental molecules of life studied at the nanoscale
The fundamental molecules of life: nucleic acids, proteins, lipids and carbohydrates are heterogeneous at the nanoscale. This structural heterogeneity determines the functionality of biomolecules and affects the ability to react with other molecules. Therefore, the chemical structure and composition of the molecules of life should also be investigated at the nanoscale preferably in physiological buffers, see Lipiec et al., Angew. Chem. Int. Ed. 2020
Our group applies molecular nanospectroscopic techniques: tip-enhanced Raman spectroscopy (TERS) and infrared nanospectroscopy supported by scanning probe microscopy (SPM) and conventional analytical tools including Raman and infrared spectroscopies. Molecular nanospectroscopy combines nanometric spatial resolution of SPM and chemical selectivity of Raman or infrared spectroscopies providing information on the chemical structure and composition of nano-volumes of investigated samples. Schematic representation of the TERS set-up is presented in Fig. 1. AFM probe modified with plasmonic nanostructures in contact with the sample surface, leads to an enhancement of the Raman scattered light. The TERS signal is generated from a very small spot, localised at the tip apex, improving the spatial resolution up to the nanoscale.
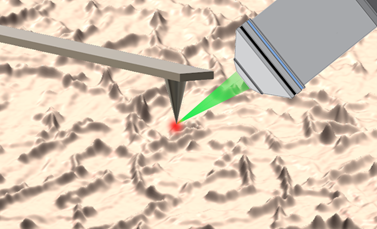
The results of our experiments deliver new knowledge about the aggregation of neurodegenerative peptides, especially specific information about the spatial arrangement of amino acids chain, i.e., a secondary structure of individual nanometric amyloid species. An example of TER spectra acquired from so called tau protein monomers (related to Alzheimer’s disease) are presented in Fig. 2. Understanding the effects of intrinsic and extrinsic factors on the secondary structure distribution, which determines the aggregation process, is essential for developing effective therapeutic strategies aimed at inhibiting self-assembly.
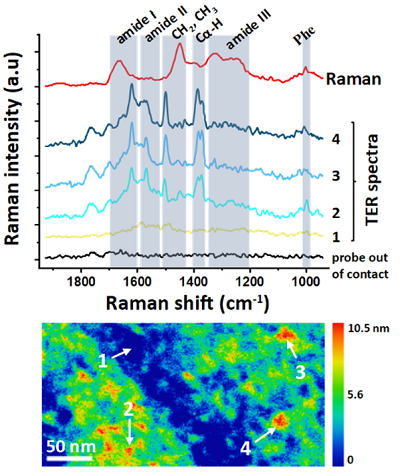
The second object of our interest is DNA molecules. We are monitoring structural changes of DNA upon damage and repair caused by anticancer drugs and other chemicals. Such studies are fundamentally important in understanding the role of DNA local conformational transitions from a so called B-DNA (right-handed, 10 base pairs per turn) to A-DNA (right-handed, 11 base pairs per turn) at the lesion sites (Fig. 3) in formation of DNA damage and DNA repair pathways. DNA conformation determines its reactivity, and in consequence DNA repair – a fundamental process, that enables continuation of life.
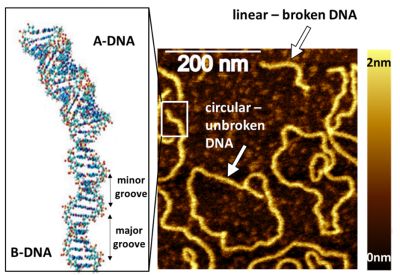
Nanomechanical profiling of dysfunctional endothelial cells and tissues
Due to its functions and location, the vascular endothelium, i.e., a tight monolayer of cells covering the inner side of the blood vessels, undergoes permanent squeezing and stretching resulting from the neighbouring environment. It is therefore apparent that knowledge of the mechanical properties of the endothelium at the cellular level is necessary for an accurate understanding of the functionalities and mechanical response of the cells to both the native and pathological environments. In the group we perform experimental investigations on the nanomechanical response of individual endothelial cells to inflammation caused by environmental agents and/or selected chemical compounds. We focus on the results obtained by means of the force spectroscopy using the tip of an atomic force microscope as an imaging and nanoindentation spectroscopic probe. The findings allow for determination of main changes in mechanical properties of the dysfunctional endothelium caused by progress of inflammation, or other pathological transformations caused by various civilization diseases, such us hyperglycemia, arteriosclerosis, and non-alcoholic fatty liver disease (see Fig.4).
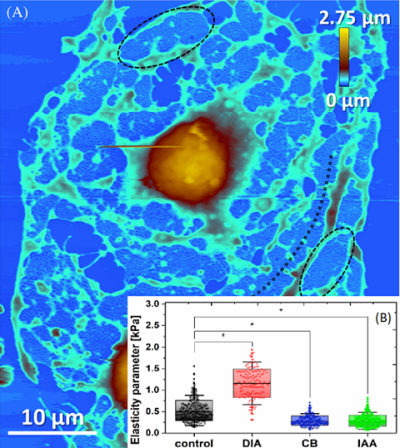
Force imaging of porous biological nanostructures
Here our group is focused on investigations of the porous structure of the liver sinusoidal endothelium (LSEC). LSEC main feature relies on the presence of fenestrae, the open membrane bound pores supported by a (sub)membranous cytoskeletal lattice grouped in sieve plates. Changes in number and diameter of fenestrae alter bidirectional transport between the sinusoidal blood and the hepatocytes. A typical size of fenestra is in the range from 50 to 300 nanometers, quite difficult for imaging in a delicate, soft materials of the live cell. Last progress in atomic force imaging allowed for fast indentation of the cellular material with a controled loading force. A high acquisition speed of force versus distance dependences during the AFM probe scanning, provides data from which both a stiffness map and a topographical image can be retrieved for a least invasive loading force. It has been shown, for the first time ever, that using such a force imaging technique the structure of fenestrae and fenestrae associated features can be resolved at the nanoscale for live LSECs in time dependent form (see Zapotoczny et al., Hepatology, 2019). Tracing live cell dynamics with force imaging AFM allows for studying time dependent changes of endothelial phenotype in response either to progressive dysfunction due to disease and/or various adverse environmental factors, or due to pharmaceutical drug treatments.
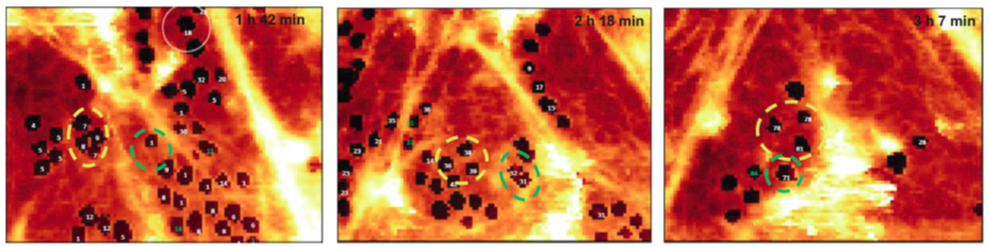
Data analysis and theoretical interpretation of the cellular nanomechanics
In our group we study the physical properties of biological systems, such as elasticity. Elasticity is considered to be an important property that is related to functional or pathological changes in cells. We concentrate on proper interpretation of the experimental data. Two most frequently used approaches are due to Hertz and Sneddon, and these are effectively one-parameter models. In our approach, we interpret the experimental force-indentation curve in a more complex way to determine an elasticity parameter, which varies with the indentation depth. We find best approximation of this property by applying the two-layer model with four effective parameters and that gives us more understanding of the complex structure of the cells and their physical properties.
Research project
Supported by:

During the implementation of OPUS 16 project, our group performed complex research on DNA structural transition at various levels of the organization (molecules, chromosomes, and cell nuclei). In particular, we have studied DNA strand breaks induced after anticancer drug (bleomycin) treatment with modern microscopical and spectroscopical techniques. Below we describe the results of our research.
Optimization of TERS probe preparation for a study of DNA molecule
The project team optimized TERS for efficient DNA measurements. We developed and tested several protocols of the probe and sample preparation procedures. Example results are presented in Fig. 6. Our research provides a solid foundation for further research on DNA and its interaction with other biomolecules upon biologically significant processes such as DNA damage and repair. For more information, please see Spectrochim. Acta A Mol. Biomol. Spectrosc. 281, 121595
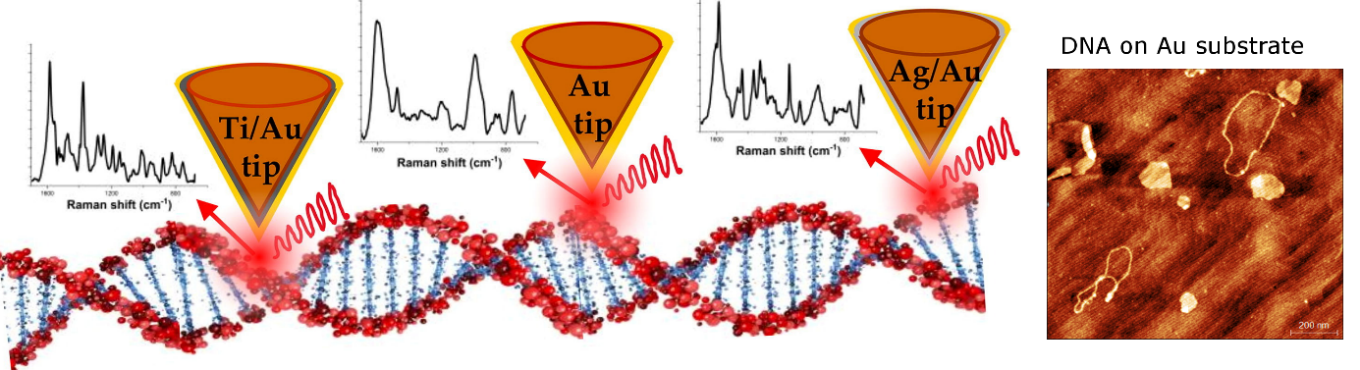
Interaction of DNA with repair proteins
We examined the dynamics of DNA mismatch repair process using the combination of atomic force microscopy (AFM) and spectroscopic techniques. AFM allowed us to visualize the structure of DNA and MutS (a protein responsible for the detection of point mutations in DNA) under simulated physiological conditions. This was possible due to the application of PeakForce Tapping mode, allowing one to visualize the delicate biological structures with the possible lowest force loading. Example images obtained in this way are shown in Fig. 7. Structural properties of DNA and MutS derived from AFM imaging enabled the proper interpretation of data obtained in other experiments, e. g. Raman and infrared spectroscopy. Also, the formation of active MutS – DNA complex was visualized using liquid-phase AFM. We have proven that dimerization of MutS protein is needed to initiate the mismatch repair process, and it is highly dependent on Mg2+ concentration. It is unambiguously shown that DNA strand breakage occurs after MutS binding, which is a crucial step needed for further mismatch proteins to finish the repair process.
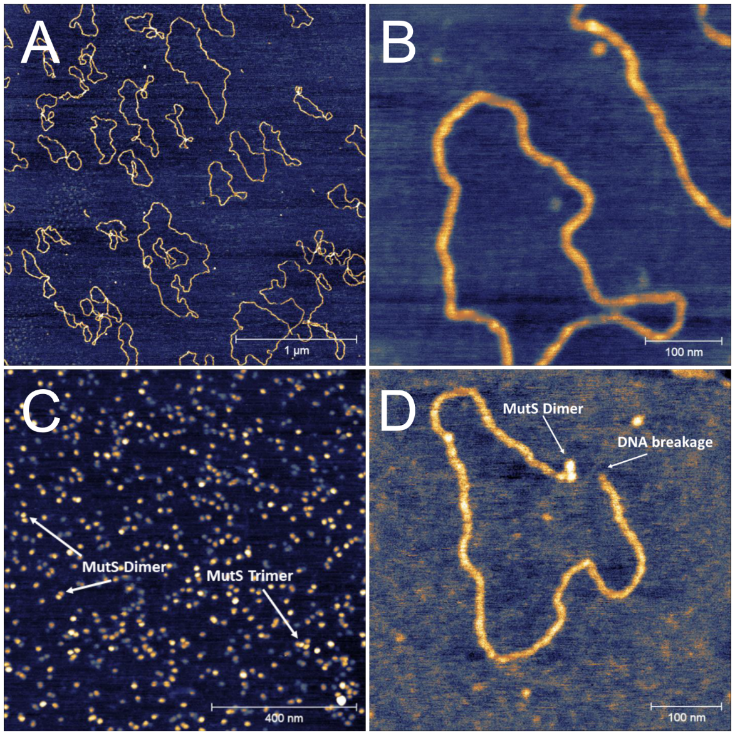
Morphological and structural changes of isolated chromosomes
Other objects of our research were chromosomes. These biological structures consist of tightly bound DNA and proteins. After treating ovarian cancer cells (HeLa line) with bleomycin, we isolated chromosomes and examined occurring changes. Bleomycin is known to cleave DNA molecules, which results in single- and double-strand breaks. If strand breaks are not repaired fast enough, it will cause alterations in chromosome structure observed as e.g. chromosomal aberrations. We used two complementary technics to investigate the effects of applied bleomycin treatment. Atomic force microscopy enabled us to observe morphological changes (see Fig. 8.), whereas Raman microspectroscopy allowed the examination of chemical alterations of chromatin structure.
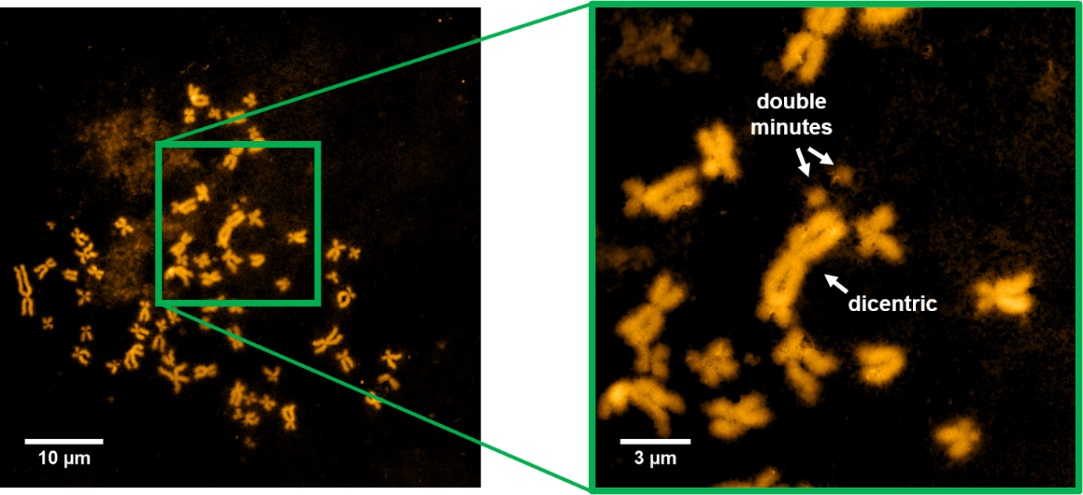
Detection of biochemical changes in living cells after induction of DNA damage
In this project, we also studied the influence of induced DNA breaks on biochemical changes in living cells. We applied Raman microspectroscopy combined with multivariate data analysis, such as Hierarchical Clustering Algorithm (HCA) and Principal Component Analysis (PCA). This enabled us to distinguish cellular regions with different biochemical fingerprints. Separated areas corresponded to various cellular organelles, including the cell nucleus (see Fig. 9.). With this approach, we were able to study systematical spectral changes related to biochemical response to treatment with various concentrations of bleomycin. These results are described in detail in Int. J. Mol. Sci. 2022, 23(7), 3524.
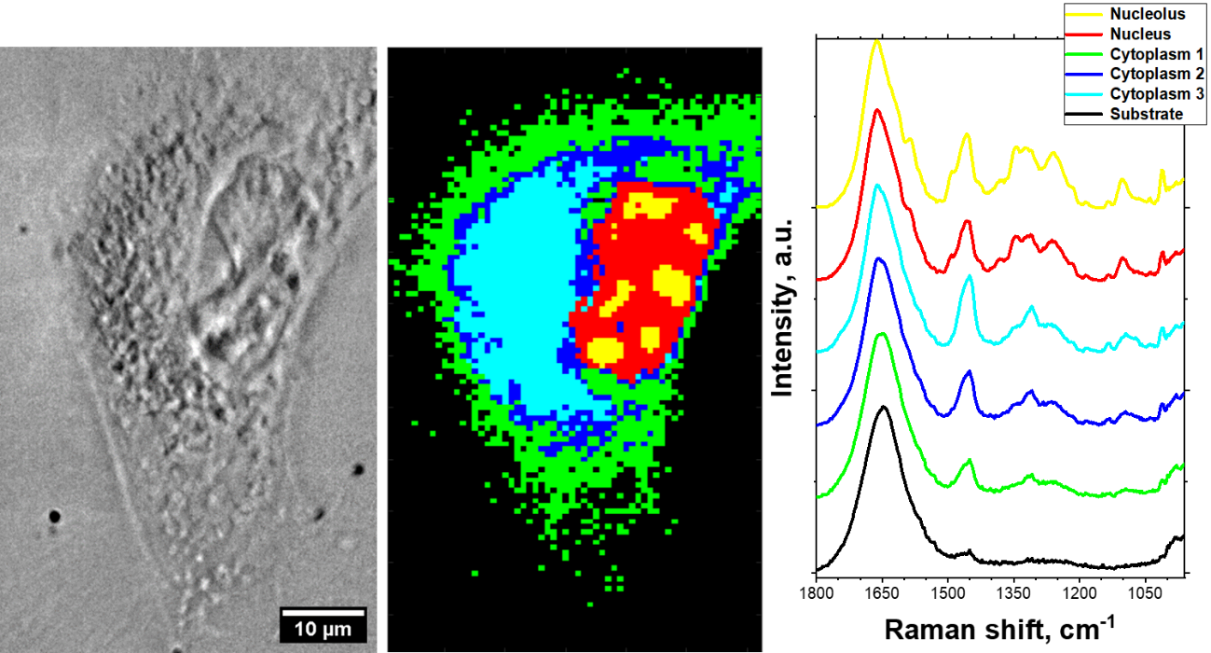
Observation of chromatin repair with fluorescence microscopy
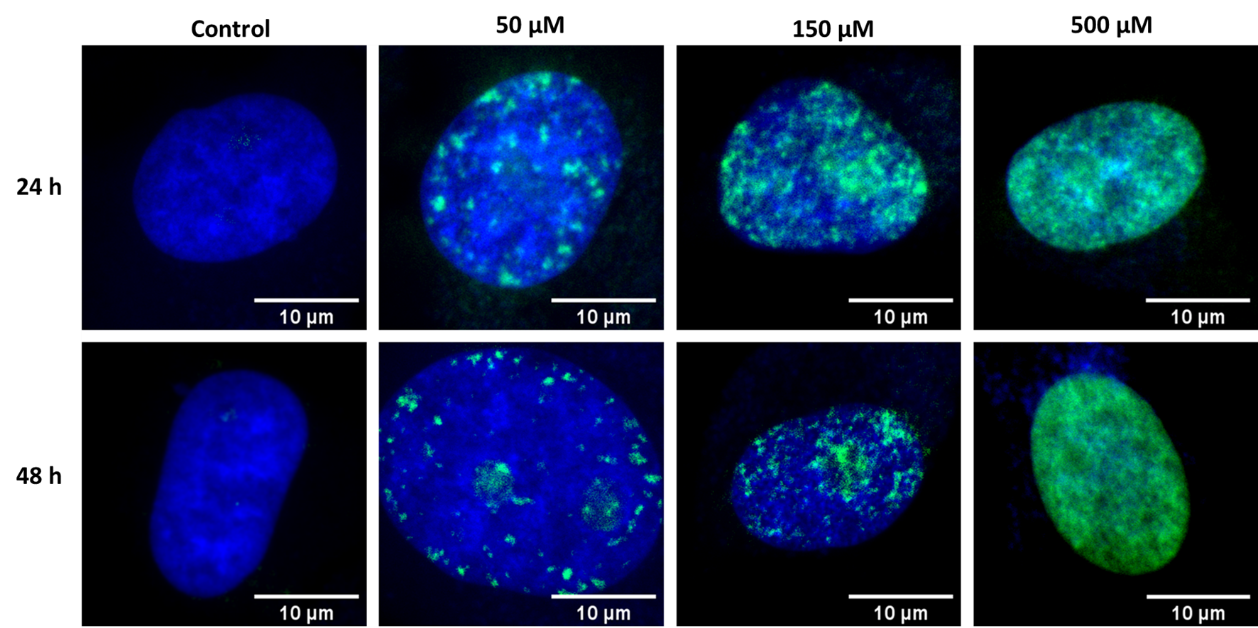
Our group applied fluorescence imaging to observe chromatin repair in HeLa cells. We used specific fluorescence dyes, which allow visualization of cell nuclei and phosphorylated histone H2A.X protein. Phosphorylation of histone H2A.X protein is a hallmark of DNA repair processes (see Fig 5.). We observed a systematic increase in the amount of damaged chromatin due to increased concentration of the applied drug. For more information, please see Int. J. Mol. Sci. 2022, 23(7), 3524
. Project team:- PhD Karol Cieślik
- MSc Michał Czaja
- PhD Ewelina Lipiec
- PhD Kamila Sofińska
- BSc Marta Urbańska
- Natalia Wilkosz
- Prof Marek Szymoński
Group members
- Dr hab. Ewelina Lipiec - Head
- Prof. dr hab. Marek Szymoński
- Dr. Dawid Lupa
- Dr. Kamila Sofińska
- Dr. Natalia Wilkosz
- Mgr Michał Czaja - PhD Student
- Mgr Sara Seweryn - PhD Student
- Mgr Katarzyna Skirlińska - PhD Student
- B.Sc. Marta Urbańska