Application of ultrathin free-standing supports in chemical analysis of ultrathin organic layers.
Duration: 2016 - 2021
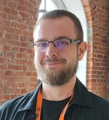
Computer simulations allow for mimicking and explaining phenomena which cannot be examined by any experimental approach. For example, some processes are so fast that it is not possible to detect them experimentally. Molecular dynamics is one of the best tools which can be used for analysis of such phenomena. However, in order to recreate reality by computer simulations a description of interactions among atoms is required. The better the description (called a force field or a potential) is, the better is the simulation in reflecting reality.
In order to increase speed of computation we will change a way of calculating electrostatic interactions. Instead of a tedious procedure of charge equilibration on each atom in a system, and explicit calculation of electrostatic interactions, we will incorporate electrostatic interaction implicitly into other terms calculating energies and forces. It will lead to a significant acceleration of computations, because algorithms connected with charges are the most time-consuming part of calculations. In order to remove the phenomenon of atoms penetrating each other during high-energy collisions we will add a barrier to the potential which will properly describe interactions at small interatomic distances.
We would like to create a potential which will accurately describe interactions among six elements, namely carbon (C), hydrogen (H), oxygen (O), nitrogen (N), sulphur (S), and phosphorus (P), which will allow for modelling structures and chemical reactions occurring in systems made of biomolecules. In order to do that, we will combine results of interatomic interactions obtained from quantum-mechanical (QM) calculations with a semi-empirical descriptions, where analytic formulae, fitted to results of QM computations, will be used to a fast prediction of forces and energies which occur in polyatomic systems.
The new potential will allow for, for example, investigating interactions between high-energy projectiles and organic or biological samples. Such phenomenon, called sputtering, is a basis of an analytic technique, called secondary ion mass spectroscopy (SIMS). This method allows for a 3D chemical imaging of samples. For example, it can detect where a drug is absorbed inside a cell, which may be used for developing new medicine faster. However, because of a lack of a proper force field, which would allow for description of interactions in such systems, it is still not understood how the SIMS works, especially when it is employed for studying organic and biologic samples. Our research will allow for modelling processes occurring in such systems. It may lead to develop more efficient analytical instruments with a better resolution, which will allow for imaging smaller systems with a higher precision.