Design of multidentate surface bonding groups as a strategy for controlling stability and conductivity of organic monolayers on metals.
Duration: 2023 - 2027
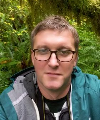
Nowadays, electronics directly or indirectly control almost every area of our lives. This situation is the result of the continuous and rapid development of this discipline, which is possible only thanks to extensive scientific research of new materials that can be used to build electronic devices. Until now, most of the materials used in electronics are inorganic materials, but gradually more and more parts of such systems are based on organic materials. In a broader perspective, this reorientation of electronics towards organic materials is well justified given that, as we all know, this type of material was evolutionarily chosen to build an extremely efficient electronic system - our brain. Although the vision of organic computers based on the so-called molecular electronics, which uses single molecules or monolayers of molecules, is only at the beginning of its development, the organic electronics devices based on the use of thick polymer layers or organic crystals are literally within our reach, taking into account the screens of smartphones or TV sets based on organic light emitting diodes (OLED) technology. Regardless whether we plan to build electronic devices based on molecular or organic electronics, we must connect the organic part of our device with metal electrodes to power and control its operation. Such a connection is quite difficult to implement both in terms of design and control of its parameters. This problem is due to the structural incompatibility of organic and inorganic materials with respect to their electronic, thermal, chemical and mechanical properties. Moreover, for devices built on a very small scale in the range of nanometers, as is the case with currently used inorganic electronic devices such as transistors, this new interface between these two types of materials becomes a very important part of our devices, which controls most of its properties.
One of the way by which such organic-inorganic interface can be created, and optimized at the molecular level, is formation of molecular monolayers called SAMs (Self-Assembled Monolayers). Importantly, as the name suggests, the formation of such monomolecular layers takes place through a spontaneous self-organization process, a process that is also responsible for building all these extremely complex biological structures that build living organisms, especially our brain. For applications in electronics the interface created by SAMs should be thermally and chemically stable, have a well-defined molecular structure (low defect concentration), specific electronic properties (e.g. conductive or insulating) and appropriate functionality, which in the case of molecular electronics should enable the connection of these layers with another metal electrode or, in the case of organic electronics, connection with a thick layer of organic semiconductor. So far most of research and applications of SAMs are based on the use of a single atom to chemically bind individual molecules to the surface of metals. To increase our control over the stability and structure of SAMs, in the present project we will study conductive and insulating monolayers formed by molecules which forms multiple chemical bonds with the metal substrate. We plan to study not only the influence of the number of such chemical bonds or the type of atom and metal surface, but most of all we will focus on the way in which such binding groups are connected to the rest of the molecule. This line of research is inspired by our recent experiences, which show that the combination of these two parts of the molecule has a very strong influence on the stability of the entire monolayer. The aim of this project is therefore to optimize the properties of SAMs that are important for their application in molecular electronics and beyond, such as defect concentration, thermal or chemical stability, conductivity, and sensitivity to electron beam irradiation. This last parameter is crucial for SAMs electron lithography reaching the nanometer scale as well as for the creation of nanometer-thick carbon membranes for the separation of gases and liquids at the molecular level. Both of these applications go well beyond molecular electronics and can be used in many other fields related to biotechnology, new energy sources and environmental protection. To sum up, we believe that the proposed research project is attractive both scientifically and technologically in a very actively developed field of nanotechnology, which stimulates the development of various areas of current science and technology.