SAMlab – Laboratory for analysis of structure, properties and application of self-assembled monolayers (SAM)
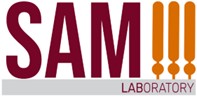
In the SAMlab laboratories, we analyze organic nanostructures produced by self-assembly (Self-Assembled Monolayers - SAM) and conduct research aimed at optimizing the structure and properties of such systems, mainly for their use in molecular and organic electronics. In our analysis, we use wide range of microscopic (STM/AFM), spectroscopic (IRRAS, XPS, TP-XPS, NEXAFS), spectrometric (SIMS, TP-SIMS) and conductivity measurements (eGaIn, C-AFM) techniques.
Why SAM and their structural optimization are important?
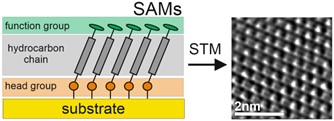
Combining inorganic and organic materials is becoming necessary for the further development of current technologies in such diverse areas as electronics, biotechnology and new energy sources. In each of these applications, this combination leads to the creation of an inorganic-organic interface, which for applications based on nanotechnology becomes one of the most critical elements of a given device. This key role is due to the profound physical and chemical differences between organic and inorganic materials, which make controlling the properties at their interface particularly difficult. Self-assembled monolayers (SAMs) provide a simple and reliable way to control the organic-inorganic interface by forming a predefined, ultra-thin, single-molecule thick organic monolayer spontaneously chemisorbed on inorganic substrates. The structure and properties of SAM are the result of the complex interaction of the three basic elements building these structures, i.e. the functional group, the head group and the hydrocarbon chain connecting these two groups (Fig. 1). Regardless of the specific application of SAMs, the most important feature of these organic nanostructures is their stability and charge transfer properties. The importance of charge transfer is rather obvious considering applications in areas such as electronics and photovoltaics, and both highly conductive and highly insulating SAM systems are of interest here. Monolayer stability, however, is actually a much more diverse property. For applications in electronics or photovoltaics, thermal stability is of key importance, taking into account both the increased temperature during the formation of a given device (e.g. deposition of organic semiconductors on the SAM surface) and the heat release during operation of the entire system. However, in biotechnology applications, thermal stability is usually of little importance considering the operation under RT conditions, however, chemical stability becomes of fundamental importance considering the competition of SAM with other adsorbates usually present in biological solutions. Moreover, for all these extensive areas of application, SAM lithography is of key importance for the spatial control of the structure and properties of the organic-inorganic interface, which is why another important aspect of SAM stability becomes crucial, i.e. stability against ionizing radiation, especially stability against the electrons beam, which provide both the highest possible spatial resolution, and allow the transformation of SAMs into other two-dimensional (2D) materials such as graphene or carbon nanomembranes (CNMs).
Selected research projects
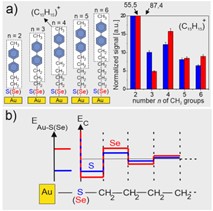
The analysis of molecule-metal interface with SIMS. As a part of this project, we demonstrated the possibility of using secondary ion mass spectrometry (SIMS) combined with density functional theory (DFT) calculations and molecular dynamics (MD) simulations to analyze the stability of chemical bonds at the molecule-metal interface. Research carried out using model SAM systems in the form of BPnS(Se)/Au(Ag) (CH3-C6H5-C6H5-(CH2)n-S(Se)/Au(Ag), the structure of which we analyzed as part of previous research recently summarized in [1]. The obtained results revealed for the first time the existence of the effect of oscillation of the stability of subsequent chemical bonds near the metal-molecule interface resulting from the breaking of translational symmetry in the molecular chain (Fig. 2). In our studies, we showed that the amplitude of this phenomenon depends on the type of group binding SAM to the metal (S/Se) as well as the selection of the metallic substrate (Au/Ag) [3]. During the implementation of this project, the possibility of using SIMS analysis as a function of sample temperature changes was also shown, which leads to the analysis of the thermal stability of the system. The conducted research revealed that the thermal and chemical stability of SAM can be inversely correlated due to the oscillation effect of chemical bond stability. In this case, this means that an increased Se-Au(Ag) binding energy compared to S-Au(Ag) is achieved at the expense of reducing the energy of the next bond, i.e. Se-C compared to S-C. While the first of analyzed bonds determines the chemical stability of the system (e.g. in exchange experiments with other molecules), the next bond is the weakest in the system and determines the range of its thermal stability.[4]
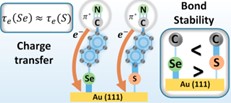
The influence of the structure and stability of self-assembled organic monolayers on the process of their electrical conductivity. The main achievement of this project was demonstration of a correlation between the stability of the chemical bond at the molecule-metal interface and the electrical conductivity of the monolayer. The measurements were carried out for a specially designed system of SAMs twin monolayers based on azobenzene, bonding to the Au surface via the S or Se atom and terminated with a nitrile group that played a double role in this experiment, i.e. enabling a full analysis of the orientation of molecules on the surface (through NEXAFS measurements for the C and N signals) and carrying out charge transfer measurements using the resonance Auger spectroscopy (RAES) technique (Fig. 3). Spectroscopic (IRRAS, XPS, NEXAFS) and microscopic (STM) measurements showed [5] that both types of monolayers form very similar structures, which provided the basis for a reliable analysis of the molecule-metal bonding stability [5], thermal stability [4] and charge transfer analysis [5]. Stability measurements based on molecular exchange revealed greater stability of the Se-Au bond compared to S-Au [5]. However, SIMS measurements [5] turned out to be crucial, as they not only confirmed this relationship but, above all, showed that increasing the Au-Se bond energy comes at the cost of reducing the energy of the neighboring Se-C bond compared to the sulfur-based system. This observation allowed to explain the surprising result of measuring the charge transfer time through the system, which, as it turned out, did not depend on the bond strength of the molecule to the substrate. Importantly, this result is contrary to the predictions made in all previous works analyzing this fundamental problem for molecular electronics. In our opinion, the lack of such a relationship is due to the fact that the increase in the binding energy to the substrate (Se-Au > S-Au) comes at the cost of reducing the energy of the neighboring chemical bond (Se-C < S-C), which in turn only leads to a redistribution of valence electron density at the molecule-metal interface and does not affect the effective change in the tunneling time through the system. Moreover, the unique combination of stability analysis (SIMS) results in these reference systems with theoretical (DFT) calculations that were performed for them, allowed us to propose the binding geometry model of molecules based on thiols and selenols on the Au(111) substrate in which bonding to the substrate takes place through additional substrate atoms (adatoms). [4]. In this project, we also analyzed the formation of SAM monolayers based on alkynes (CH3-(CH2)n-CC-Au) in which carbon plays the role of the bonding group to the metal surface.
Our research revealed for the first time that these compounds form highly ordered monolayers with the structure which is very similar to that known for classic SAM monolayers based on alkanethiols.[6] Conductivity measurements have shown that despite the much greater stability of the Au-C bond compared to Au-S, the conductivity of monolayers based on alkynes is, within the limits of accuracy, similar to monolayers based on thiols, regardless of whether we analyze aliphatic or aromatic compounds.[7, 8] This project also analyzed biologically inspired SAM monolayers based on peptides and ethylene glycol, revealing surprisingly high electrical conductivity of these systems, which indicates the possibility of their use in organic electronics.[9,10]
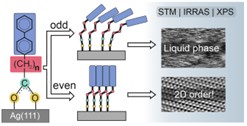
SAMS based on a carboxyl binding group. In this project, we analyzed (STM/IRRAS/XPS) the possibility of using the carboxyl bonding group to form SAM monolayers and their application in molecular electronics and to form 2D materials by interacting with the electron beam. In the first stage of the project, by comparing chemically and structurally analogous monolayers of BP2COO/Ag BP2COO/Ag (CH3-C6H4-C6H4-(CH2)2-COO/Ag) and BP2S/Ag (C6H4-C6H4-(CH2)2-S/Ag), we showed that replacing the binding group from thiol to carboxyl allows for an approximately 30-fold increase in the size of domain structures and, therefore, an analogous level of reduction in defect concentration, with a simultaneous 300-fold reduction in the monolayer formation time.[11] The analysis of the homologous series of BP2COO/Ag (n = 1-6) revealed a very strong parity effect occurring in those monolayers in which crystalline ordered structures are formed for even values of the parameter n, suitable for molecular electronics applications requiring the lowest possible concentration of defects, while for odd values, a disordered ("liquid") structure is formed, suitable for use in the field of biotechnology, where the SAM monolayer should be able to fit into amorphous biological structures (Fig. 4). [12] Our research has shown that this structural parity effect has a direct impact on the efficiency and course of the modification process of these monolayers by irradiation with a low-energy (50 eV) electron beam [13], and that for properly selected monolayers this process leads to the formation of carbon nanomembranes (CNMs), which are free from sulfur contamination.[14] In the next stage of this project, we analyzed the impact of changing the binding group (from S or Se to COO) on the electrical conductivity, showing that this change is not significant and that when using naphthalene-based monolayers, exceptionally highly conductive SAM monolayers with high thermal stability can be fabricated, thus very well suited for organic electronics applications.[15]
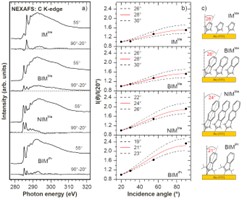
SAMS based on carbenes. In this project, we mainly analyzed the possibility of forming and using SAM monolayers based on N-heterocyclic carbenes (NHC) deposited on a gold surface by forming a C-Au bond. Our research revealed that, contrary to previous experiments and predictions, vertically oriented monolayers can be formed using NHC molecules in which the side groups of nitrogen atoms forming the NHC structure are small (Fig. 5). Reducing the size of the side groups obviously affects the surface area occupied by the NHC molecules in the monolayer, and thus their packing density. Our comprehensive spectroscopic analysis (XPS/NEXAFS) using a series of NHC molecules showed that in the case of fabricating monolayers from solution, such a modification of the structure of NHC molecules allows them to maintain their vertical orientation, leading to a doubling of the packing density compared to typical NHC monolayers [16, 17].
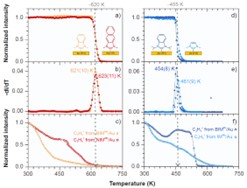
These studies also revealed (Fig. 6) that the appropriate design of the structure of NHC molecules through the use of small side groups and the selected structure of the core of the molecule allows the creation of SAMs on the gold surface with record high thermal stability (desorption energy of 1.9 eV).[17] Moreover, considering that the molecules forming such ultra-stable monolayers are terminated with an aromatic or naphthalene ring, their application in the field of molecular electronics is possible. This range of applications is interesting not only due to the high thermal stability of NHC systems but also due to their conductivity properties. Our studies indicate that the conductivity of these monolayers is approximately 4 orders of magnitude lower than that of alkanethiol molecules, which are considered highly insulating.[16] This means that these monolayers can be a very interesting alternative to (i) forming ultra-thin layers insulating the organic semiconductor from the gate electrode in OFET type transistors or (ii) in the case of using a longer aromatic core, constitute an active element of a SAMFET type transistor, i.e. a system in which the SAM monolayer has low conductivity in the direction perpendicular to the metallic substrate on which it is formed (providing thus insulation from the gate electrode) and high conductivity in a direction parallel to the substrate (ensuring efficient transport along the channel connecting the source and drain electrodes).
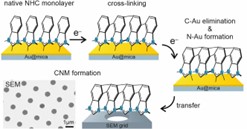
In this project, we also analyze for the first time electron irradiation of NHC SAMs, using a series of monolayers with different numbers of benzene groups and different sizes of side nitrogen groups to modify their packing density and chemical stability (Fig. 7).[18] Changes in the thickness and composition of the monolayer were analyzed (XPS) as a function of electron dose. Our results provide simple design rules for optimizing the structure of NHC SAMs for their efficient modification by the electron beam. Such optimization becomes particularly interesting considering that the analyzed NHC monolayers show up to 100 times higher stability of their bond with the metal substrate against electron irradiation compared to standard thiols or carboxylic acids. Thus, NHC monolayers offer a new and exciting alternative to chemical lithography in which structural modification of the SAM with electron beams or photons (X-ray, UV, or EUV-induced SAM damage is actually due to photoelectrons and secondary electrons) should be limited mainly to the end group, keeping intact bond with the substrate. Furthermore, we demonstrate successful delamination and transfer of electron-irradiated NHC monolayers, which allows the formation of carbon nanomembranes (CNMs) (Fig. 7). As we show, this process is also very sensitive to the structure of the NHC SAM and, with a properly designed system, allows the creation of continuous, free-standing CNMs that do not contain sulfur and are therefore much more suitable for certain ultra-filtration applications compared to standard CNMs produced from thiols , which are contaminated with reactive sulfur.
Formation of SAM monolayers on the surface of natural aluminum oxide. This project is focused on the optimization of the structure of SAM monolayers created on aluminum, a much more available and widely used material in the field of electronics and medicine. Our research in this project is mainly inspired by the use of SAM for functionalization of surface-oxidized aluminum working as a gate electrode in organic field-effect transistors (OFET). The performance of these devices is based on the degree of crystallinity of the organic semiconductor (OSC) in the conduction channel area (increased mobility of charge carriers), the quality of the insulator and the proper charge distribution at the gate-OSC interface (reduced leakage currents). Improving all of these parameters requires the creation of high-quality, well-defined SAMs with the lowest possible defect concentration. By examining key parameters for carboxylic SAM preparation, such as solvent type, incubation time and incubation temperature, and using a combination of several complementary experimental techniques (NEXAFS/XPS/IRRAS/AFM), we proposed a set of parameters enabling preparation of densely packed and well-ordered aliphatic (insulating) SAMs with carboxylic head group on naturally oxidized aluminum.[19] Our structural analysis shows that, contrary to popular opinion, the highest quality carboxyl-based monolayers are obtained using "bad" solvents and a relatively low incubation temperature, which can be explained by the intercalation of solvent molecules in the SAM matrix. Moreover, we have shown that the naturally formed AlOx top layer (x ~ 1.38) with a thickness of ~ 0.8 nm creates a dipole layer, which, similarly to dedicated SAM monolayers, allows changing the work function of the metal electrode on which it is formed. In the next step, we carried out analogous structural studies for monolayers in which we replaced the carboxylic group with a phosphonic one (Fig. 8).
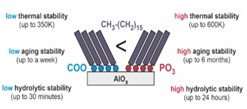
Our analysis shows that the quality of the monolayers produced for a properly selected solvent is as good as in the case of model monolayers of thiols on the gold surface. These studies also shows that the hydrolytic stability of structurally optimized phosphonic SAMs on AlOx is approximately 50 times higher compared to analogous carboxylic SAMs. In turn, aging studies in laboratory conditions showed the stability of phosphonic SAMs on AlOx for up to 6 months, compared to only a week's stability of analogous carboxyls. In this project, we also showed that the thermal stability limit of structurally optimized phosphonic SAMs on AlOx exceeds the stability of analogous carboxylic monolayers by about 250 K, and that of standard alkanethiol monolayers on gold by about 200 K. Taking into account the significant heat dissipation in organic electronics systems as well as the increased temperature at which they are produced (e.g. during the deposition of an organic semiconductor onto a metal electrode), very high thermal stability makes phosphonic SAM monolayers on AlOx an excellent candidates for use in organic electronics.
Selected publications
[1] Cyganik et al. Nano Res. 2024, 17, 4231. [2] Ossowski et al. Angew. Chem. Int. Ed. 2015, 54, 1336. [3] Ossowski et al. J. Phys. Chem. C 2017, 121, 459. [4] Ossowski et al. J. Phys. Chem. C 2017, 121, 28031. [5] Ossowski et al. ACS Nano 2015, 9, 4508. [6] Żaba et al. J. Am. Chem. Soc. 2014, 136, 11918 [7] Bowers et al. ACS Nano 2015, 9, 1471. [8] Bowers et al. J. Phys. Chem. C 2016, 120, 11331. [9] Baghbanzadeh et al. Angew. Chem. Int. Ed. 2015,127, 14956. [10] Baghbanzadeh et al. J. Am. Chem. Soc. 2017, 139,7624. [11] Krzykawska et al. Chem. Comm. 2017, 53, 5748. [12] Krzykawska et al. J. Phys. Chem. C 2018, 122, 919. [13] Kruk et al. J. Phys. Chem. C 2021, 125, 9310. [14] Neumann et al. ACS Appl. Mater. Inter. 2019, 11, 31176. [15] Wróbel et al. Adv. Electron. Mater. 2021, 7, 2000947. [16] Krzykawska et al. ACS Nano 2020, 14, 6043. [17] Wróbel et al. Nano Today 2023, 53, 102024. [18] Cegiełka et al. J. Phys. Chem. Lett. 2024, 15, 8196. [19] Cegiełka et al. Appl. Surf. Sci. 2023, 636, 157798. [20] Cegiełka et al. Appl. Surf. Sci. 2024, 665, 160199.Collaborators
- Prof. Michael Zharnikov, Heidelberg University, Germany
- Prof. George Whitesides, Harvard University, USA
- Prof. Andrey Turchanin, Friedrich Schiller University Jena, Germany
- Prof. Mariusz Krawiec, Marie-Curie University, Lublin, Poland
- Prof. Egbert Zojer, TU Graz, Austria
Group members
- Prof. dr hab. Piotr Cyganik - Head
- Dr. Mateusz Wróbel - Assistant
- Mgr Daria Cegiełka - PhD Student
- Mgr Agnieszka Grabarek - PhD Student
- Małgorzata Lebica - Master student
- Mark Kasatkin - Student
- Karolina Sorn - Student
- Marta Szatny - Student
Former group members
- Dr. Maciej Dendzik
- Dr. Joanna Dudek (Sobczuk)
- Mgr Dominika Gnatek
- Dr. Monika Kruk (Szwed)
- Dr. Anna Krzykawska
- Mgr Małgorzata Niemiec
- Mgr Agnieszka Noworolska
- Dr. Jakub Ossowski
- Mgr Katarzyna Szelągowska
- Dr. Agnieszka Szpak
- Dr. Tomasz Żaba