Nanocrystals for energy conversion
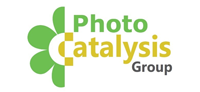
Solar irradiation provides a cheap and abundant source of energy which could cover the whole energy needs of mankind. However, it is highly intermittent, weather dependent and subject to daily and annual cycles. It needs to be stored for later use, preferably in a form that it can be extracted from with ease.
The group explores the means to convert incident solar energy into fuel, such as methane, methanol, or hydrogen. It uses the energy to drive energetically up-hill reactions that take water and carbon dioxide and result in the energy-dense products that can be combusted at will to recover the stored energy.
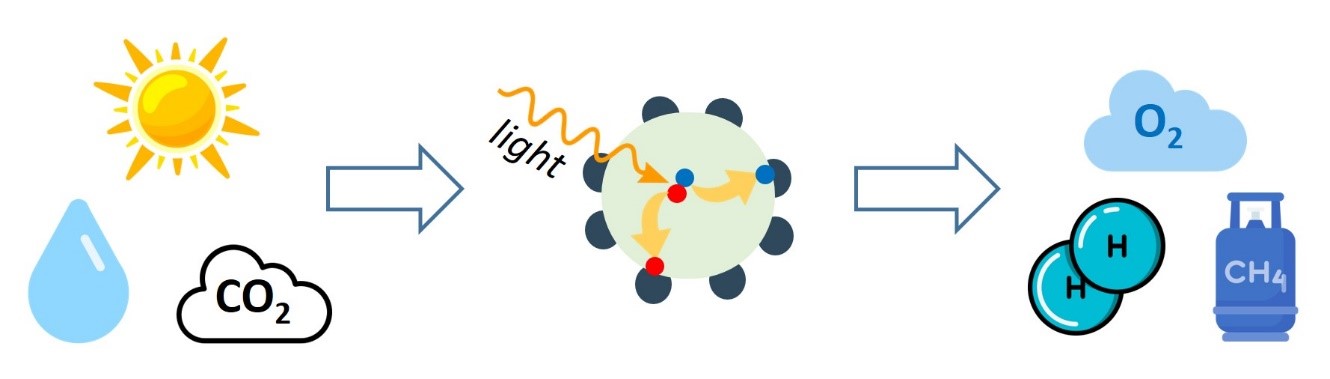
How is it done? We use semiconductor nanocrystals, that is tiny nanometer-size particles, which absorb the solar light, generate electron-hole pairs that part their ways and transfer to separate catalytic sites at the particle surface. There, the charge carriers induce chemical reduction and oxidation reactions, respectively, which result in converting water and/or CO2 into hydrogen, oxygen and several useful carbon-containing products.
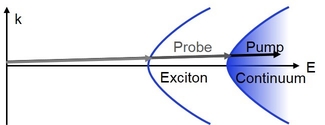
It may sound straightforward, but in reality, it requires a highly complex series of physical processes that proceed at vastly different time scales, ranging from femtoseconds to microseconds, to occur exactly when needed and exactly where needed. It also needs to outcompete various parallel, parasitic or backward processes that recombine the charges or waste them to create undesired or useless products. To overcome them and make the energy conversion process efficient, the mechanism needs to be understood. This way the slowest or least efficient steps can be targeted for improvement or circumvented altogether. To this end, we use methods of time-resolved absorption and emission spectroscopy, primarily in the picosecond to microsecond regime, to investigate the dynamics of the charge carriers.
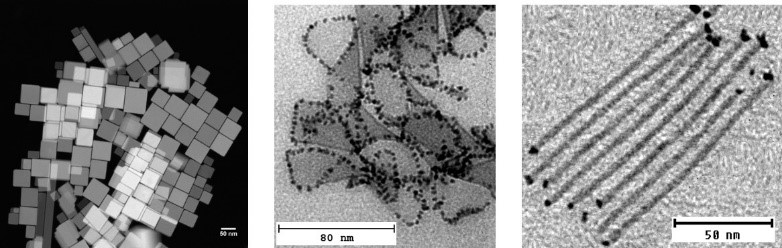
Nanocrystals make an excellent tool to achieve this. They are incredibly versatile in size, shape, form, and composition so that their geometric, optoelectronic, and catalytic properties can be tuned to the task in question. We work with several types of nanocrystals for which their morphology or inherent properties break the symmetry for the dynamics of electrons and holes. This facilitates their separation and enables to maintain the separation for long enough for the desired chemical reactions to happen.
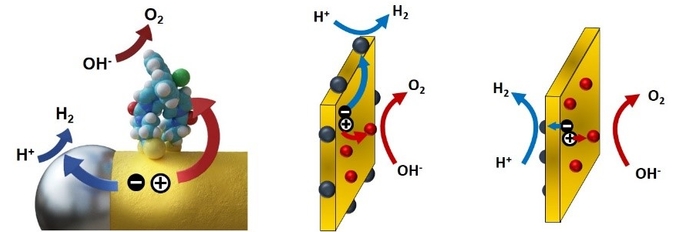
Two examples of charge separation facilitated by anisotropy are shown above. On the left-hand side, CdS nanorod decorated selectively with reduction and oxidation catalysts at the tips and side surfaces, respectively, can split water into hydrogen and oxygen (see ref. [8]). On the right-hand side, nanoplatelets offer several ways to separate the charges and reduction and oxidation sites.
Selected publications
- S. Habisreutinger, L. Schmidt-Mende, J. K. Stolarczyk, Photocatalytic CO2 reduction on TiO2 and other semiconductors”, Angew. Chem. Int. Ed. 2013, 52, 7372-7408
- T. Simon, N. Bouchonville, M. J. Berr, A. Vaneski, A. Adrović, D. Volbers, R. Wyrwich, M. Döblinger, A. S. Susha, A. L. Rogach, F. Jäckel, J. K. Stolarczyk, J. Feldmann, Redox shuttle mechanism enhances photocatalytic H2 generation on Ni-decorated CdS nanorods, Nature Mater. 2014, 13, 1013-1018
- J. A. Sichert, Y. Tong, N. Mutz, M. Vollmer, S. F. Fischer, K. Z. Milowska, R. Garcia Cortadella, B. Nickel, C. Cardenas-Daw, J. K. Stolarczyk, A. S. Urban, J. Feldmann, Quantum Size Effect in Organometal Halide Perovskite Nanoplatelets, Nano Lett. 2015, 15, 6521–6527
- J. K. Stolarczyk, A. Deak, D. F. Brougham, Nanoparticle clusters: assembly and control over internal order, current capabilities and future potential, Adv. Mater. 2016, 28, 5400-5424.
- T. Simon, M. T. Carlson, J. K. Stolarczyk, J. Feldmann, Electron Transfer Rate vs Recombination Losses in Photocatalytic H2 Generation on Pt-decorated CdS Nanorods, ACS Energy Lett. 2016, 1, 1137-1142.
- S. Bhattacharyya, F. Ehrat, P. Urban, R. Wyrwich, M. Döblinger, J. Feldmann, A. S. Urban, J. K. Stolarczyk, Effect of Nitrogen Atom-Positioning on the Trade-off between Emissive and Photocatalytic Properties of Carbon Dots, Nature Commun. 2017, 8, 1401.
- F. Ehrat, S. Bhattacharyya, J. Schneider, A. Löf, R. Wyrwich, A. L. Rogach, J. K. Stolarczyk, A. S. Urban, J. Feldmann, Tracking the Source of Carbon Dot Photoluminescence: Aromatic Domains versus Molecular Fluorophores, Nano Letters 2017, 17, 7710–7716
- C. M. Wolff, P. D. Frischmann, M. Schulze, B. J. Bohn, R. Wein, P. Livadas, M. T. Carlson, F. Jäckel, J. Feldmann, F. Würthner, J. K. Stolarczyk, Visible-light-driven water splitting using CdS decorated with nanoparticulate and molecular co-catalysts, Nature Energy 2018, 3, 862-869
- J. Fang, T. Debnath, S. Bhattacharyya, M. Döblinger, J. Feldmann, J. K. Stolarczyk, Just-in-time delivery: Photobase effect in photocatalytic hydrogen generation, Nature Communications 2020 11, 5179
- S. Rieger, T. Fürmann, J. K. Stolarczyk, J. Feldmann, Optically Induced Coherent Phonons in Bismuth Oxyiodide (BiOI) Nanoplatelets, Nano Letters, 2021, 21, 7887–7893
Group members
- Dr hab. Jacek Stolarczyk, prof. UJ